Design Strategy for Cell Penetrating Peptides
Introduction
Cell-penetrating peptides (CPPs) have the ability to penetrate biological membranes and drive cells to internalize a bioactive payload. CPPs, also known as protein transduction domains (PTDs), are small peptides with <30 amino acids in length. The first reported CPPs were derived from the Tat peptide (positions 48-60) of the HIV-1 transcriptional protein and penetratin from the amphipathic Drosophila Antennapedia homeodomain.
The CPP database was established in 2012 and currently has been updated to CPP version 2.0, providing useful information. There are 1699 distinct CPP sequences, according to the statistics section of the CPP database site. Of these, the majority are linear CPPs (94.5%), which are employed to transport fluorophores (58.4%). The most of the studies on CPPs are synthetic peptides (54.8%) that are based on 84.3% of l-amino acids.
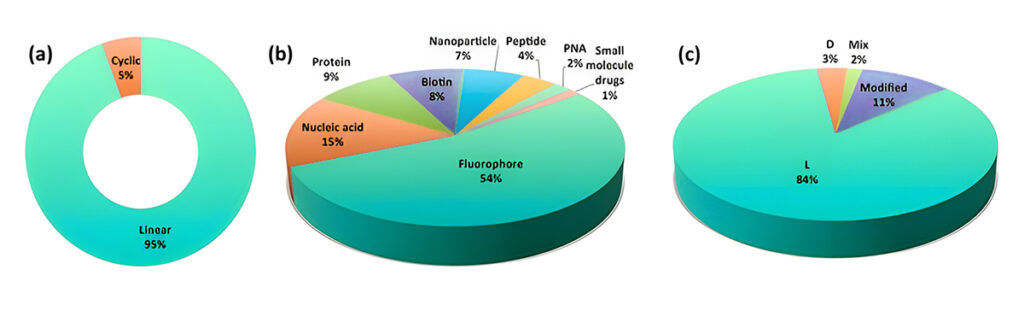
Important Primary Sequence
Charge, guanidine, hydrophobicity, and aromaticity are important drivers of CPP internalization, and their combinations are frequently examined to produce more effective CPPs. The contributions of charge, hydrophobic functionality, π-electronics and helicity are controlled through a recently developed CPP synthetic mimetic (CPPM). These mimetics were created by ring-opening metathesis polymerization, which produced polypeptides with a variety of interest functions.
Cationic and Arginine-Rich CPPs
In the field of CPP, peptide sequences with high content of cationic amino acid side chain show tremendous promise. Various polycationic compounds containing repeating arginine residue scaffolds that promote cell penetration have attracted attention. The guanidinium group is related to membrane translocation, because it can create bidentate hydrogen bonds with membranes. Synthetic mimics that containing guanidinium moieties or other positively charged chemical entities, as well as non-natural amino acids (d-stereoisomers) have also been investigated.
Cell-Penetrating Polydisulfides
Cell-permeable polysulfides (CPD) are a conceptually innovative approach to increasing cell permeability. The peptide backbone of polydisulfide, which are guanidinium-rich molecules formed by ring-opening disulfide-exchange polymerization, is replaced by disulfide bonds. CPD participates in dynamic covalent chemistry on the cell surface and enters cells via thiol-mediated uptake as shown below.
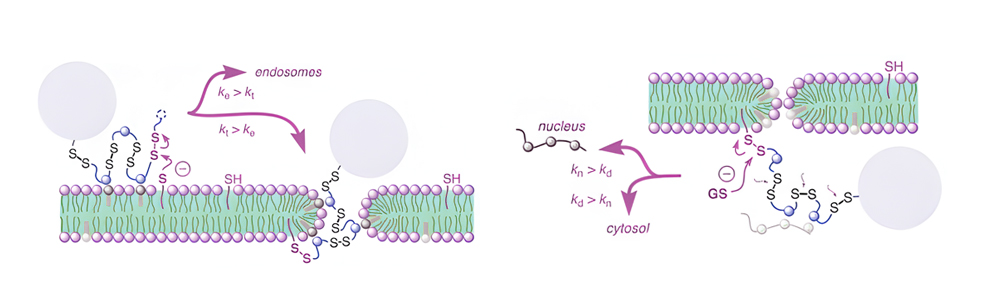
The CPDs displayed in the picture are the most promising. During cellular internalization, they are reductively depolymerized by glutathione, and endosomes cannot trap them, so they are advantageous.
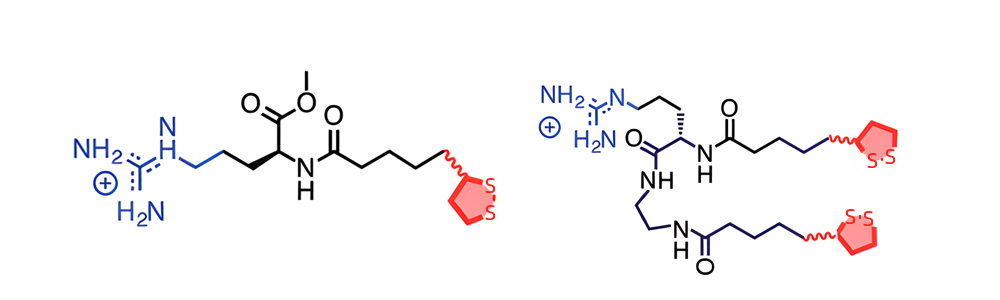
The Role of Hydrophobicity and Aromaticity
Transmembrane complexes can be formed by the hydrophobic counterions. You can make a substance hydrophobic via adding aromatic or aliphatic molecules. One method of achieving lipidation is by joining hydrocarbon chains of various lengths to the N-terminal of recognized CPPs. It is also possible to the peptide sequence are incorporated into amino acids with alkyl side chains to introduce hydrophobicity. An illustration of CPP mimetics, which replicate the hydrophobic counterion action by combine guanidinium with aliphatic groups of different lengths from methyl to dodecyl, to increase internalization efficiency.
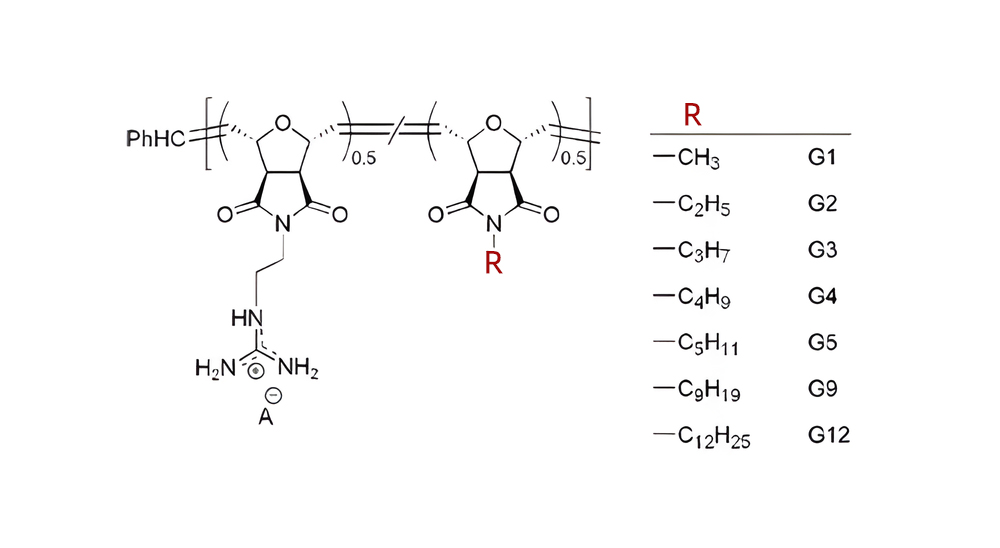
Another approach is to add aromatic amino acid residues to achieve hydrophobicity, such as tryptophan, phenylalanine, and tyrosine. Guanindine-containing CPP mimetics introduce aromatic functionality including octyl, cyclohexyl, phenyl, naphthyl, and pyrenyl.
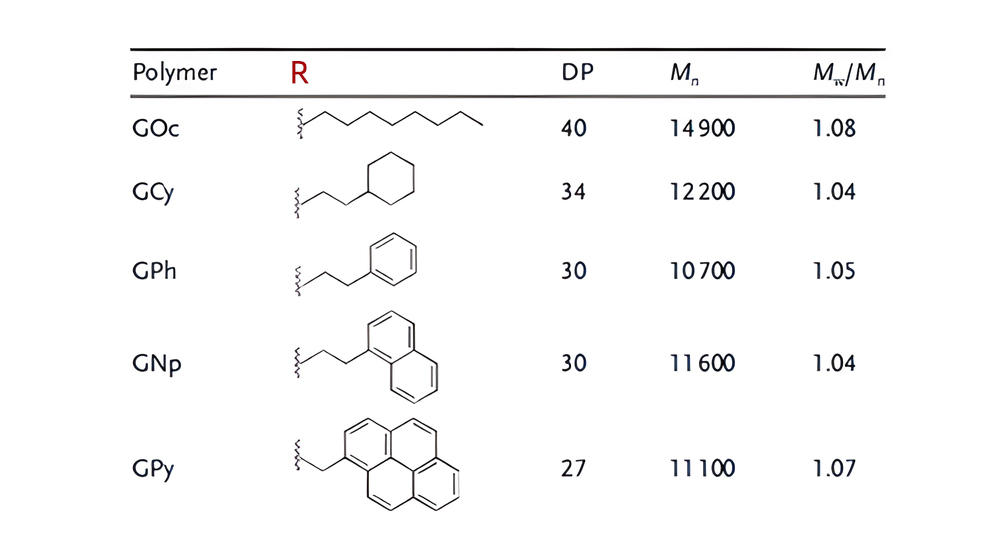
Chirality
One of the main obstacles to the use of conventional CPPs in vivo based on natural L-amino acids is their susceptibility to proteases. The primary structure of CPPs has been designed with a number of stability-enhancing strategies. These mostly rely on the usage of non-natural amino acids, including l- and d-combinations and all d-stereoisomer forms of CPPs. Although the exact role of chirality in internalization efficiency is yet unknown, proteolysis resistance is the primary benefit of employing d-stereoisomers in CPP design.
Flexible Amphipathic CPPs
Upon coming into contact with cell membranes, well-known CPPs as Pep-1, MPG, TP10, and SAP have been demonstrated to either assume helical conformation or adopt intrinsic helical structures. Membrane permeation is promoted by their folded state. Cell-penetration roperties are exhibited by polyproline helices PPII helices.
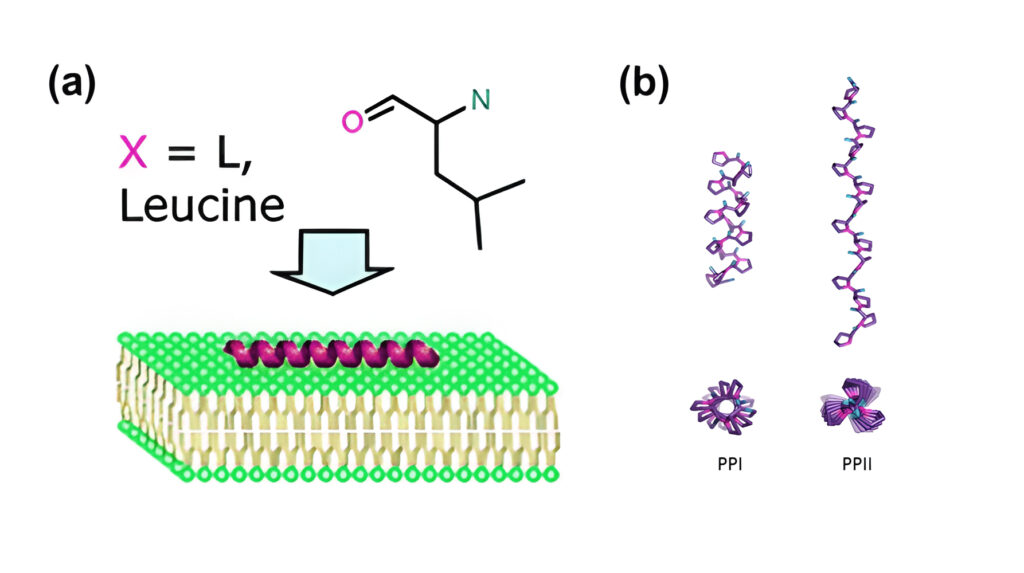
Folding and Secondary Structure of CPPs
When examining how secondary structures affect CPP translocation efficiency, two key factors need to be taken into account: (1) the affinity of CPPs for membranes, (2) their ability to fold in the presence of membranes. Due to their shared foundation in weak interactions, these two factors are related. Their participation is crucial to comprehend the factors that propel CPP internalization and to enhance the design of CPP in the future.
Designing Sequences for Helicity Induction or Stabilization
It is possible to achieve helicity in primary sequence design by employing a naturally occurring, non-coded α-aminoisobutyric acid (Aib). Numerous amphipathic nonapeptides with achiral Aib residues, d-Arg, and l-Arg were developed by researchers. They noticed that he helical structure was beneficial to the cross membranes ability of peptide. The peptides, which formed stable α-helical structures, were more effectively absorbed into Hela cells than non-helical ones.
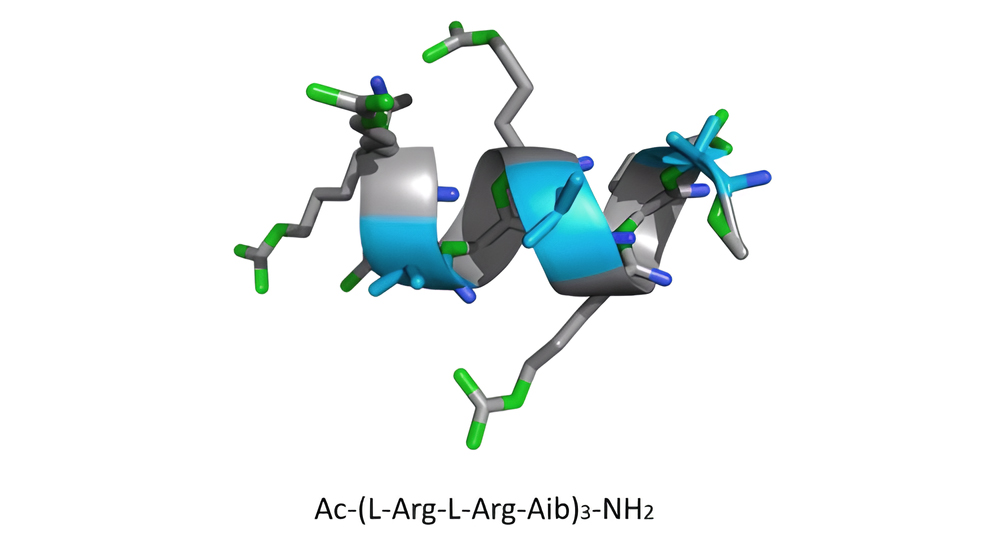
The image below depicts the relationship between CPP’s secondary structure properties and cell permeability in various environments.
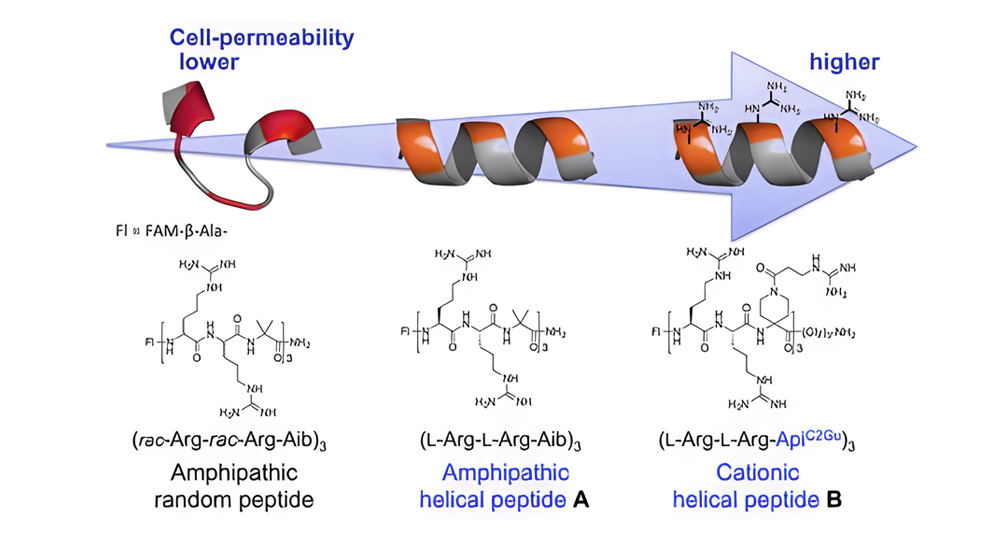
Rigidity Concept in CPP Design
One means of maximizing membrane contacts is cyclization, which has been demonstrated to improve the kinetics of Tat internalization (cyclic Tat vs. linear Tat). Furthermore, multivalency has been achieved through the dimerization and transient (reversible) cyclization of di-sulfide bonds or triazole bridges.
Head-to-Tail Cyclization
(1) Design of cyclic CPPs that contain different structural domains via repeating arginine units, hydrophobic region that promote membrane interactions and target-binding area.
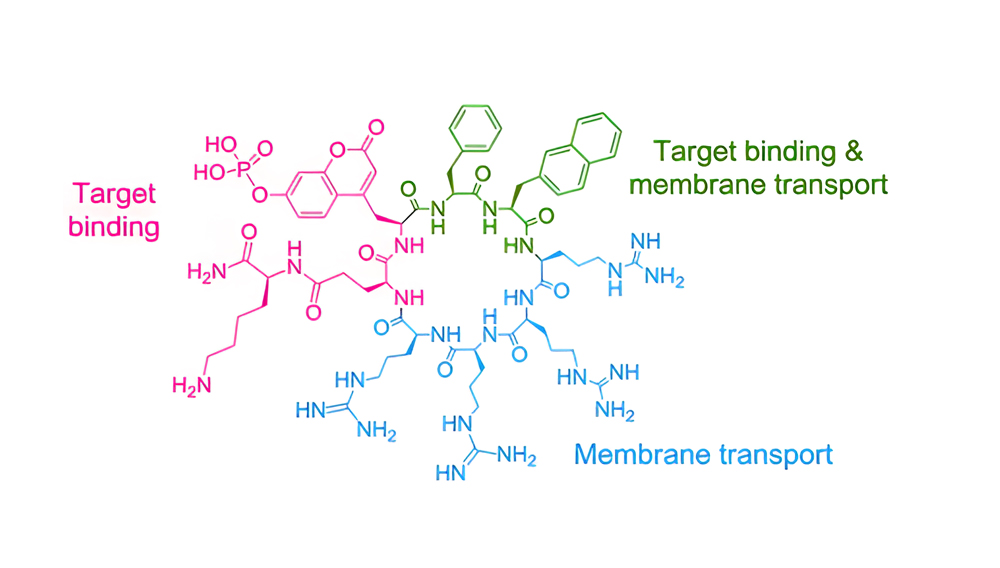
(2) Different cyclic CPPs were designed based on the alternation of arginine with tryptophan residues, in which the [WR]4 candidate showing the best performance.
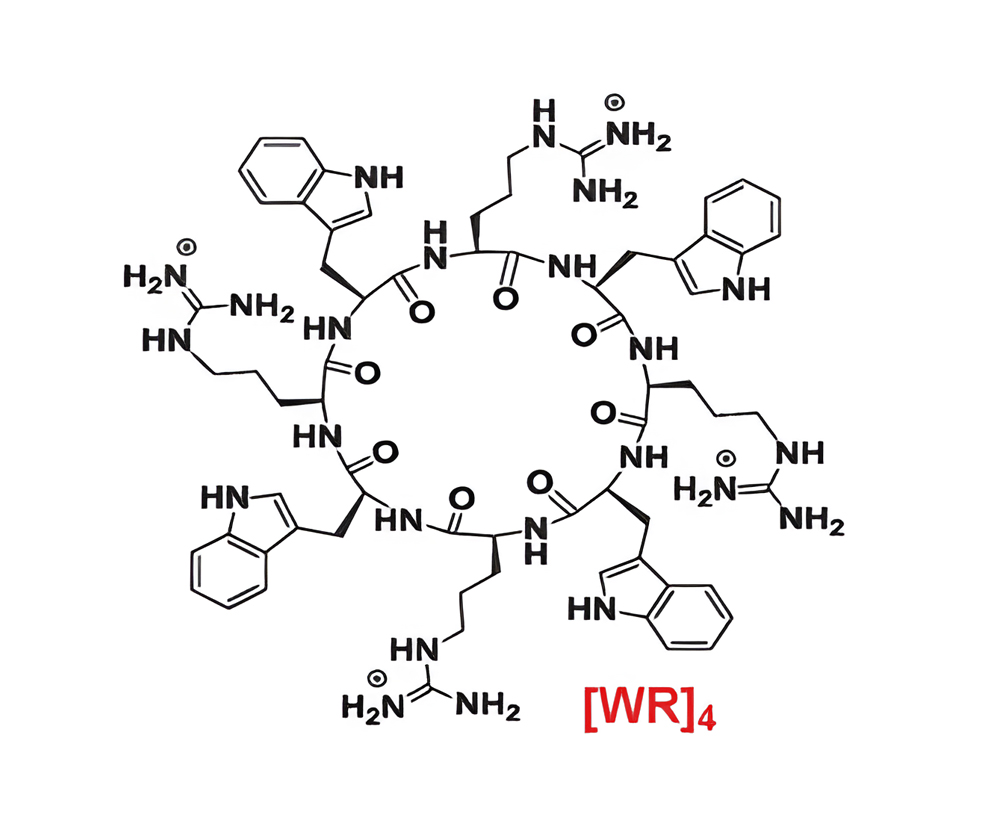
(3) Utilizing a combinatorial library method, cyclic peptides with enhanced cell permeability were found.
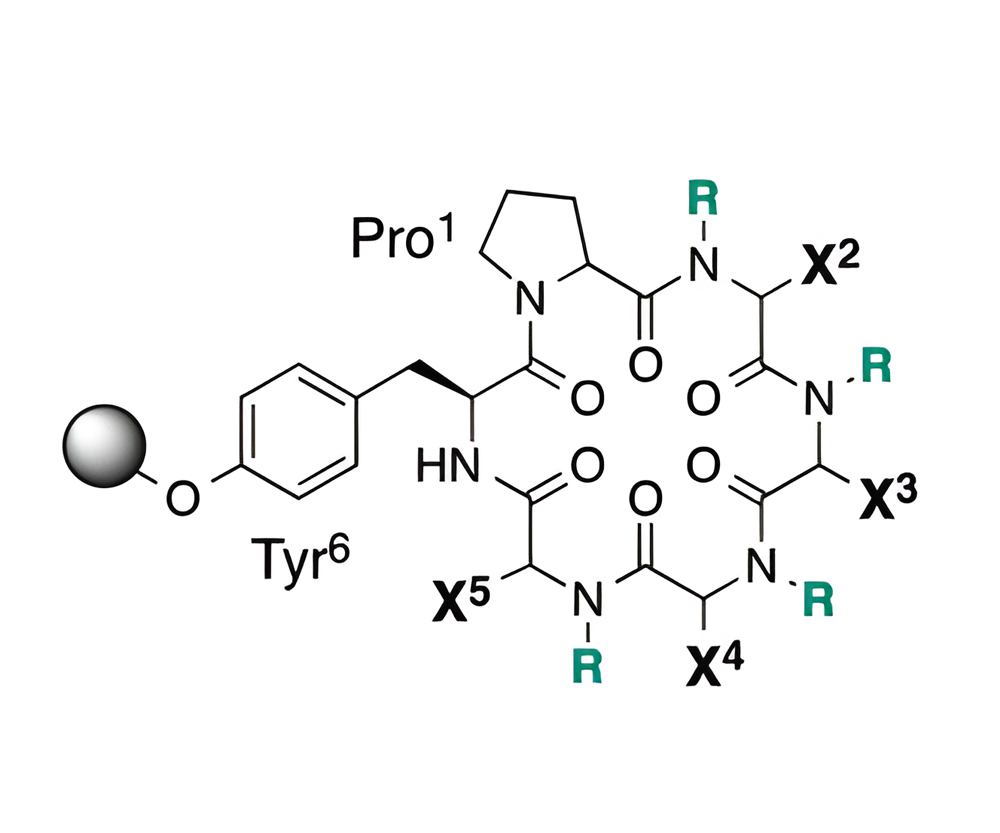
Disulfide Bridges and Bicyclization
(1) An intramolecular di-sulfide cyclization was formed by modifying the sequence to contain two cysteine residues.
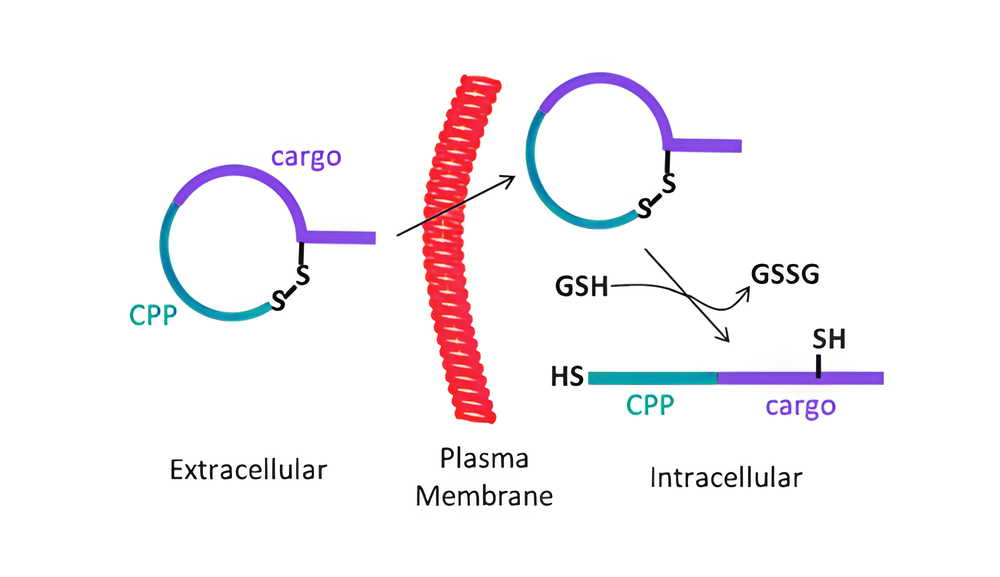
(2) To improve the uptake of cyclic peptides that are normally impermeable, a bicyclization approach was suggested.
(3) The combinatorial library technique was proposed for bicyclic CPPs, combining cFΦR4 with over 5 million different cyclic peptides to create a bicyclic cell-permeable peptide library.
(4) By forming a pair of disulfide bonds, a reversible bicyclization strategy is achieved.
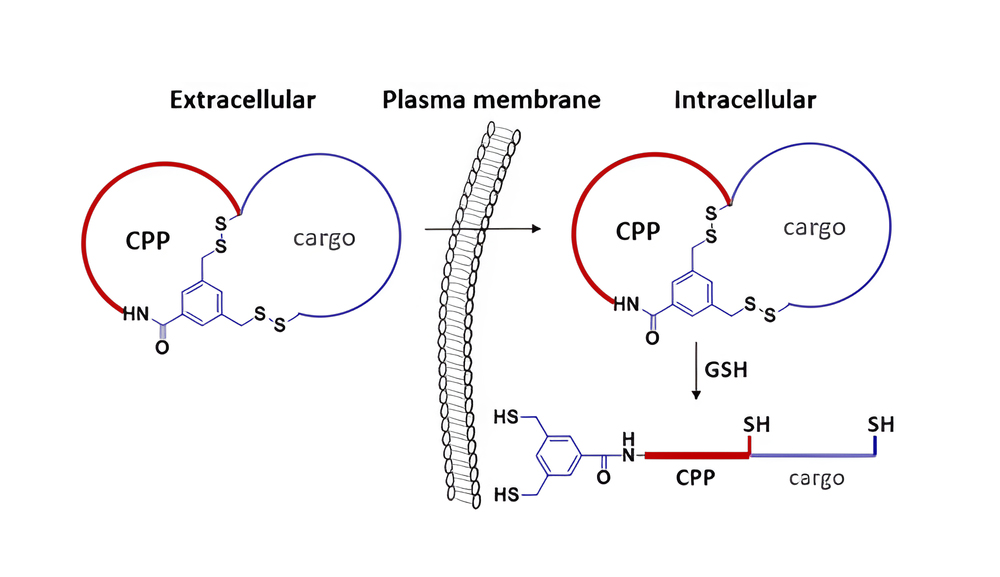
Multivalency
The multivalency of the system is also a parameter to be considered. The development of dimeric branched peptides is a step in this direction. The branched peptide with covalent dimer was created as a Tat analogue. Near the C-terminus, bis-Fmoc protected lysine allowed for controlled dimerization.
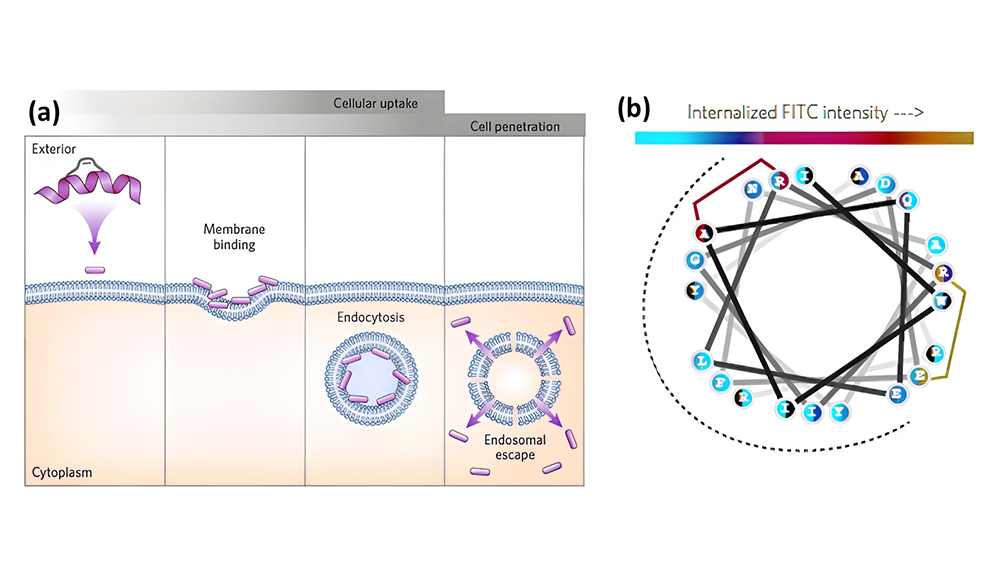
Conformational restriction
The formation of covalent bonds between two side chains of short peptides enables to obtain stabilized helices, including disulfide bonds, stapling, and N-terminal aspartic acid crosslinking.
Controlled Self-assembly
(1) Main chain or templated are the two primary methods available for achieving multivalency in self-assembled systems.
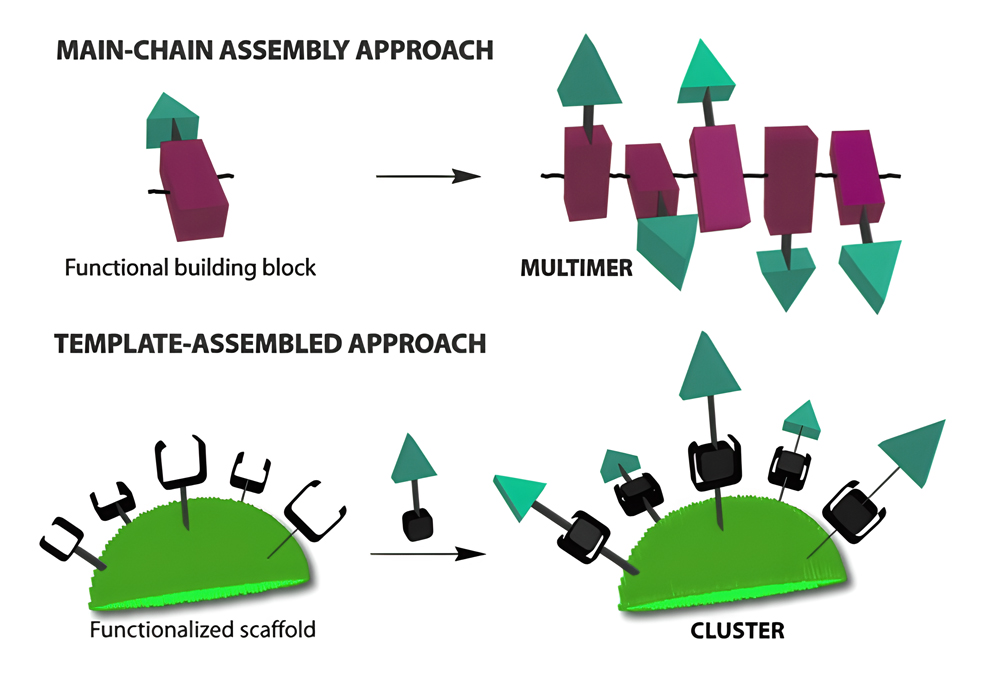
(2) One-dimensional supramolecular structures that precise length and tunable characteristics can be created by utilizing DNA as a template. With careful design, these nanostructures have the potential to achieve cell permeability, and also can transport a variety of cargos.
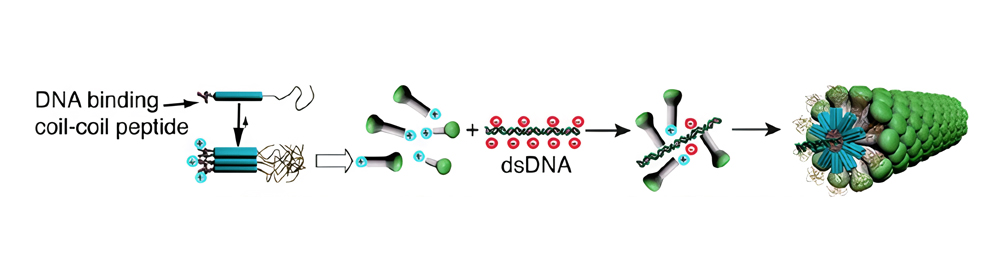
(3) Supramolecular, enzyme-responsive CPP amphiphile undergo fiber-to-micelle transition by MMP-2 triggered. In addition, the exposure of their CPP moieties leads to entry cells.
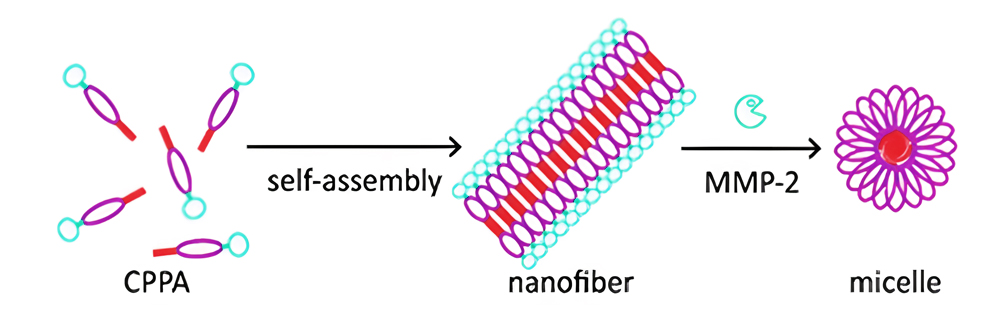
Conclusion
The design strategies of CPP are diverse, which allow to control over folding, cyclization, dimerization, stapling, self-assembly and even CPP mimetics with different backbone structures and properties, thus improving internalization efficiency and stability. QYAOBIO can design and synthesize a variety of CPPs.